These Living Computers Are Made from Human Neurons
These Living Computers Are Made from Human NeuronsIn the search for less energy-hungry artificial intelligence, some scientists are exploring living computersBy Jordan KinardFour clusters of living neurons are connected to electrodes on FinalSpark’s Neuroplatform chip. Artificial intelligence systems, even those as sophisticated as ChatGPT, depend on the same silicon-based hardware that has been the bedrock of computing since the 1950s. But what if computers could be molded from living biological matter? Some researchers in academia and the commercial sector, wary of AI’s ballooning demands for data storage and energy, are focusing on a growing field known as biocomputing. This approach uses synthetic biology, such as miniature clusters of lab-grown cells called organoids, to create computer architecture. Biocomputing pioneers include Swiss company FinalSpark, which earlier this year debuted its “Neuroplatform”—a computer platform powered by human-brain organoids—that scientists can rent over the Internet for $500 a month.“As far as I know, we are the only ones in the world doing this” on a publicly rentable platform, says FinalSpark co-founder Fred Jordan. Initially bankrolled with funds from its co-founders’ previous start-up, FinalSpark seeks an environmentally sustainable way to support AI. “Our principal goal is artificial intelligence for 100,000 times less energy” than what’s currently required to train state-of-the-art generative AI, Jordan says. Neuroplatform uses a series of processing units hosting four spherical brain organoids each. Every 0.5-millimeter-wide organoid is connected to eight electrodes that electrically stimulate the neurons within the living sphere; those electrodes also link the organoids to conventional computer networks. The neurons are selectively exposed to the feel-good neurotransmitter dopamine to mimic the human brain’s natural reward system. These twin setups—positive dopamine rewards and electrical stimulation—train the organoids’ neurons, prompting them to form new pathways and connections much in the same way a living human brain appears to learn. If perfected, this training could eventually allow organoids to mimic silicon-based AI and serve as processing units with functions similar to today’s CPUs (central processing units) and GPUs (graphics processing units), FinalSpark says.For now, the organoids and their behavior are live streamed 24 hours a day for researchers (and anyone else) to observe. “The challenge is to find the appropriate way to get neurons to do what we want them to do,” Jordan says.On supporting science journalismIf you're enjoying this article, consider supporting our award-winning journalism by subscribing. By purchasing a subscription you are helping to ensure the future of impactful stories about the discoveries and ideas shaping our world today.One of FinalSpark’s neural organoids, magnified nearly 1,000 times, made of 10,000 living neurons taken from human skin.Research teams at 34 universities have asked to use FinalSpark’s biocomputers, and so far the company has provided access for scientists at the University of Michigan, the Free University of Berlin and seven other institutions. Each one’s project focuses on a different aspect of biocomputing. The University of Michigan team, for example, is investigating the electrical and chemical prompts necessary to change organoid activity—in effect creating the building blocks of an organoid-specific computer language. Scientists at Lancaster University Leipzig in Germany, meanwhile, are trying to fit the organoids into different models of AI learning.Sticking points remain for organoid computing’s ability to compete with silicon on a large scale. For one thing, no standardized manufacturing system exists. And living brains die: FinalSpark’s organoids only survive for an average of around 100 days (and that’s considerable progress from the original experiment’s lifespan, which was just a few hours). But Jordan notes that Neuroplatform has “streamlined” its in-house process for making organoids, and its facility currently houses between 2,000 and 3,000 of them.FinalSpark is not alone in its pursuit of organic alternatives to silicon-based computing, and brain organoids are not the only possible way forward. “There are different flavors of biocomputing,” says Ángel Goñi-Moreno, a researcher at Spain’s National Center for Biotechnology. Goñi-Moreno studies cellular computing, or the use of modified living cells to create systems that can replicate “memory, logic gates and the other decision-making basics we know from conventional computer science,” he says. His team is looking for tasks at which biocomputers outperform their silicon counterparts—a dynamic he calls “cellular supremacy.” In particular, Goñi-Moreno believes that because cellular computers can react to their environmental conditions, they could facilitate bioremediation, or the restoration of damaged ecosystems. “That’s a domain where conventional computers can do basically nothing,” Goñi-Moreno says. “You can’t just throw a computer into a lake and have it tell you the state of the environment.” A submerged bacterial computer, however, would be able to give a nuanced reading of environmental conditions as the cells respond to chemical and other stimuli.Where Goñi-Moreno is focused on bacteria, Andrew Adamatzky of the University of the West of England, founding editor in chief of the International Journal of Unconventional Computing, has been studying the computational possibilities of fungus. Mycelia, or networks of fungal strands, exhibit spiking electrical potentials similar to those found in neurons, Adamatzky says. He hopes to take advantage of these electrical properties to create a brainlike fungal computing system that is “potentially capable of learning, reservoir computing, pattern recognition, and more.” Adamatzky’s team has already successfully trained fungal networks to help computer systems perform certain mathematical functions. “Fungal computing offers several advantages over brain-organoid-based computing,” Adamatzky says, “particularly in terms of ethical simplicity, ease of cultivation, environmental resilience, cost-effectiveness and integration with existing technologies.”Jordan is well aware of the considerations involved in using cultivated human neurons for nonmedical purposes. An ongoing bioethical debate concerns whether mini brains could gain consciousness, though there is as yet no evidence it has ever been created in a lab. Jordan says he is currently seeking philosophers and researchers with the “cultural background to help us answer these ethical questions.”Adamatzky acknowledges that brain organoids “might offer advanced functionalities due to their complex and neuronlike structures” despite his advocacy of fungal computing. Jordan, for his part, is confident in FinalSpark's choice for its biocomputers. Of all the cells to pick from, he says, “human neurons are the best at learning.”
In the search for less energy-hungry artificial intelligence, some scientists are exploring living computers
These Living Computers Are Made from Human Neurons
In the search for less energy-hungry artificial intelligence, some scientists are exploring living computers
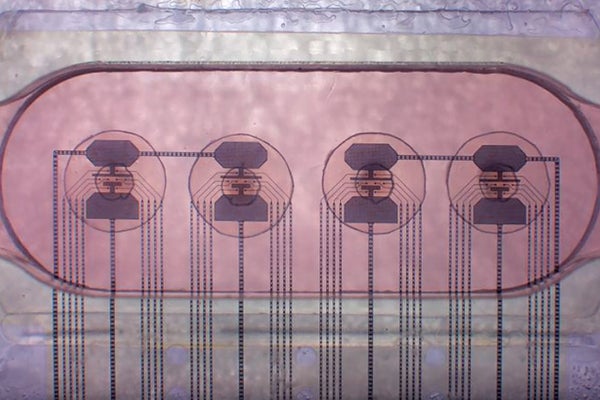
Four clusters of living neurons are connected to electrodes on FinalSpark’s Neuroplatform chip.
Artificial intelligence systems, even those as sophisticated as ChatGPT, depend on the same silicon-based hardware that has been the bedrock of computing since the 1950s. But what if computers could be molded from living biological matter? Some researchers in academia and the commercial sector, wary of AI’s ballooning demands for data storage and energy, are focusing on a growing field known as biocomputing. This approach uses synthetic biology, such as miniature clusters of lab-grown cells called organoids, to create computer architecture. Biocomputing pioneers include Swiss company FinalSpark, which earlier this year debuted its “Neuroplatform”—a computer platform powered by human-brain organoids—that scientists can rent over the Internet for $500 a month.
“As far as I know, we are the only ones in the world doing this” on a publicly rentable platform, says FinalSpark co-founder Fred Jordan. Initially bankrolled with funds from its co-founders’ previous start-up, FinalSpark seeks an environmentally sustainable way to support AI. “Our principal goal is artificial intelligence for 100,000 times less energy” than what’s currently required to train state-of-the-art generative AI, Jordan says. Neuroplatform uses a series of processing units hosting four spherical brain organoids each. Every 0.5-millimeter-wide organoid is connected to eight electrodes that electrically stimulate the neurons within the living sphere; those electrodes also link the organoids to conventional computer networks. The neurons are selectively exposed to the feel-good neurotransmitter dopamine to mimic the human brain’s natural reward system. These twin setups—positive dopamine rewards and electrical stimulation—train the organoids’ neurons, prompting them to form new pathways and connections much in the same way a living human brain appears to learn. If perfected, this training could eventually allow organoids to mimic silicon-based AI and serve as processing units with functions similar to today’s CPUs (central processing units) and GPUs (graphics processing units), FinalSpark says.
For now, the organoids and their behavior are live streamed 24 hours a day for researchers (and anyone else) to observe. “The challenge is to find the appropriate way to get neurons to do what we want them to do,” Jordan says.
On supporting science journalism
If you're enjoying this article, consider supporting our award-winning journalism by subscribing. By purchasing a subscription you are helping to ensure the future of impactful stories about the discoveries and ideas shaping our world today.
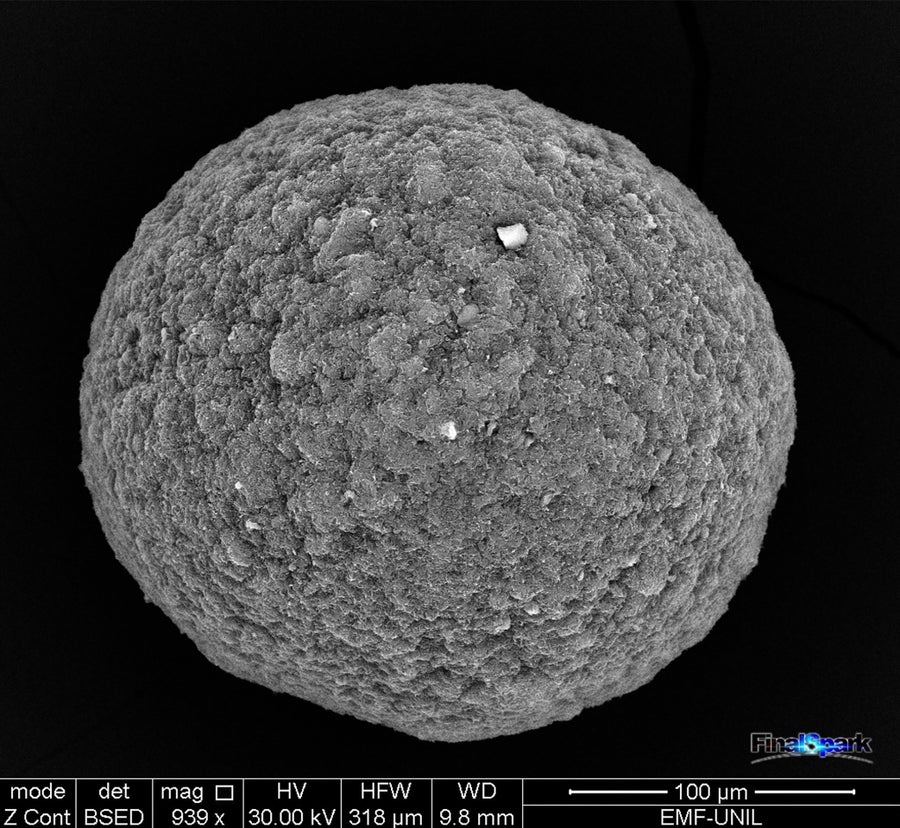
One of FinalSpark’s neural organoids, magnified nearly 1,000 times, made of 10,000 living neurons taken from human skin.
Research teams at 34 universities have asked to use FinalSpark’s biocomputers, and so far the company has provided access for scientists at the University of Michigan, the Free University of Berlin and seven other institutions. Each one’s project focuses on a different aspect of biocomputing. The University of Michigan team, for example, is investigating the electrical and chemical prompts necessary to change organoid activity—in effect creating the building blocks of an organoid-specific computer language. Scientists at Lancaster University Leipzig in Germany, meanwhile, are trying to fit the organoids into different models of AI learning.
Sticking points remain for organoid computing’s ability to compete with silicon on a large scale. For one thing, no standardized manufacturing system exists. And living brains die: FinalSpark’s organoids only survive for an average of around 100 days (and that’s considerable progress from the original experiment’s lifespan, which was just a few hours). But Jordan notes that Neuroplatform has “streamlined” its in-house process for making organoids, and its facility currently houses between 2,000 and 3,000 of them.
FinalSpark is not alone in its pursuit of organic alternatives to silicon-based computing, and brain organoids are not the only possible way forward. “There are different flavors of biocomputing,” says Ángel Goñi-Moreno, a researcher at Spain’s National Center for Biotechnology. Goñi-Moreno studies cellular computing, or the use of modified living cells to create systems that can replicate “memory, logic gates and the other decision-making basics we know from conventional computer science,” he says. His team is looking for tasks at which biocomputers outperform their silicon counterparts—a dynamic he calls “cellular supremacy.” In particular, Goñi-Moreno believes that because cellular computers can react to their environmental conditions, they could facilitate bioremediation, or the restoration of damaged ecosystems. “That’s a domain where conventional computers can do basically nothing,” Goñi-Moreno says. “You can’t just throw a computer into a lake and have it tell you the state of the environment.” A submerged bacterial computer, however, would be able to give a nuanced reading of environmental conditions as the cells respond to chemical and other stimuli.
Where Goñi-Moreno is focused on bacteria, Andrew Adamatzky of the University of the West of England, founding editor in chief of the International Journal of Unconventional Computing, has been studying the computational possibilities of fungus. Mycelia, or networks of fungal strands, exhibit spiking electrical potentials similar to those found in neurons, Adamatzky says. He hopes to take advantage of these electrical properties to create a brainlike fungal computing system that is “potentially capable of learning, reservoir computing, pattern recognition, and more.” Adamatzky’s team has already successfully trained fungal networks to help computer systems perform certain mathematical functions. “Fungal computing offers several advantages over brain-organoid-based computing,” Adamatzky says, “particularly in terms of ethical simplicity, ease of cultivation, environmental resilience, cost-effectiveness and integration with existing technologies.”
Jordan is well aware of the considerations involved in using cultivated human neurons for nonmedical purposes. An ongoing bioethical debate concerns whether mini brains could gain consciousness, though there is as yet no evidence it has ever been created in a lab. Jordan says he is currently seeking philosophers and researchers with the “cultural background to help us answer these ethical questions.”
Adamatzky acknowledges that brain organoids “might offer advanced functionalities due to their complex and neuronlike structures” despite his advocacy of fungal computing. Jordan, for his part, is confident in FinalSpark's choice for its biocomputers. Of all the cells to pick from, he says, “human neurons are the best at learning.”